The successful, long-term performance of orthopedic implants depends on: implant material, prosthesis design, biocompatibility of the component, wear of the articular surfaces, quality of the bone, and stability of fixation.
Long-term stability of fixation in bone can be achieved with either biological or cemented anchorage. Biological anchorage is achieved by ongrowth or ingrowth of bone, thus inducing an intimate contact of the tissue to the (structured) bioactive or, at least, bioinert surfaces. Cemented anchorage achieves fixation with the help of a form-fitting cement that fills the gaps between the implant and the inner surface of the trabecular bone.
Cementing with self-curing substances was the first, and initially the only, technique to achieve a stable fixation of the implants. Themistokles Gluck, a German surgeon, was the first surgeon to implant a total knee replacement made of ivory.
He applied a mixture of plaster and colophony for cemented fixation of his ivory total knee arthroplasties in 1870. He fixed the stems in both the tibia and the femur with cement: “…for a better fixation, I mixed plaster with colophony, which cures up to the hardness of glass.”1
Today, the use of polymethylmethacrylate (PMMA) bone cement is a widely used method of implant fixation. This fixation technique largely contributes to the success of modern joint replacement.
Polymethylmethacrylate as a polymer is commonly used in daily life. Plexiglas, for example, is an acrylic resin that was invented at the beginning of the 20th century and, at that time, manufactured under complex technical conditions. In 1936, cold curing of methylmethacrylate (MMA) was developed and introduced into dentistry and craniofacial surgery.
Sir John Charnley,2,3 who is considered the founder of modern artificial joint replacement, developed the science and art of modern cementing technique in his laboratories in the late 1950s. Despite a variety of cementless prostheses, cement fixation remains the gold standard, against which all forms of implant fixation techniques are assessed.
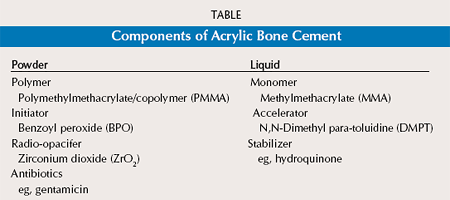
Composition of Bone Cement
Bone cements are usually supplied as two component systems (Table) made up of liquid and powder. The powder mainly consists of bead-shaped particles with a diameter typically of 40 microns.
These particles contain, in addition to homopolymer PMMA and/or methyl methacrylate copolymers, one of the three activators of the polymerization process, benzoyl peroxide (BPO) (so called initiator), zirconia (ZrO2), or barium sulphate (BaSO4) to provide radiodensity, and an antibiotic, which is, in most cases, aminoglycoside gentamicin.
The liquid as the second component mainly contains the monomer MMA but also the second activator of the polymerization process, N,N-Dimethyl para-toluidine (DMPT) (so called accelerator), and hydroquinone as a stabilizer to prevent self-curing of the monomer in the liquid during storage. In scanning electron microscopic views of cured PMMA probes, it is possible to detect all components of bone cement (Figures 2).
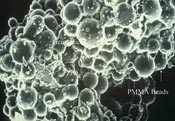
Polymerization
When both components are mixed, the polymerization process is initiated and self-curing occurs. At room temperature, the polymerization of the monomer can be activated only in the presence of free radicals. Radicals are atoms or groups of atoms with at least one unpaired electron.
Radicals are considered highly reactive and are normally destroyed quickly, either by recombination or by breaking up to create another radical and a stable molecule. These radicals are produced during the reaction of both the initiator BPO, which is in the powder, and the accelerator DMPT, which is in the liquid.
For this reason, this special type of polymerization is called free radical polymerization. The polymerization process is an exothermic reaction, which means it produces heat and is conversely affected by the application of heat. Stem temperatures of 40°-46° C have been measured on the surface of a cemented hip.
This is close to the coagulation temperature of proteins.4,5 Once polymerization ends, the temperature decreases and the cement starts to shrink. Two percent to 6% of the monomers remain and are almost totally exhaled by the lungs or metabolized in the so called citric acid cycle. Kirschner6 found 0.5% of monomers remaining in clinical retrievals of bone cement. Monomers are neither responsible for aseptic loosening nor for any cardiovascular or toxic reactions.
This has been proven by extended experimental and clinical investigations by Schlag et al.7 The initiator and accelerator are not totally consumed during the polymerization process. Benzoyl peroxide reacts to benzoate compounds, and DMPT either becomes monomethyl-p-toluidine or remains unchanged among the polymer chains. There is a harmless release of DMPT of <0.002>2per day. This concentration is unable to induce any irreversible damage to the surrounding bone at a cellular level.
Handling
Testing the phases of cement. When the dough does not stick to the latex of the gloves anymore, the working time begins. The sticky cement on the thumb of the surgeon’s right hand occurred during waiting time.
For the routine practical application of bone cement during surgery, the polymerization process is divided into four phases: mixing, waiting, working, and hardening. Handling properties of bone cements are tested according to International Standard Organization (ISO) 5833. The mixing phase starts with the addition of the liquid to the powder and ends when the dough is homogenous and stirring becomes effortless.
The dough is subsequently tested with gloved fingers every 5 seconds, using a different part of the glove on another part of the cement surface on each occasion. This provides an indication of the end of the waiting phase when the cement is neither “sticky” nor “hairy.” The working phase is then initiated. This phase is finished when the cement does not join without folds during continuous kneading by hand. At this time, an implant can no longer be inserted. The hardening phase is the last period, during which the cement cures to a hard consistency. During this phase, the temperature reaches its peak.8
Only the mixing phase is regarded as remaining constant; the waiting, working, and hardening phases are dependent on the ambient temperature. The higher the temperature, the shorter the phases. Instructive package inserts provide graphic information on the duration of each period related to temperature (Figure 3).
It would be convenient for the surgical team and advantageous for intrusion of the cement into the bone if there was a relatively long working phase with a constant medium viscosity. This has been almost perfectly achieved in the SmartSet GHV gentamicin bone cement (DePuy Orthopaedics Inc., Warsaw, Ind) by introducing two copolymers, each with a different increase in viscosity during the working phase.
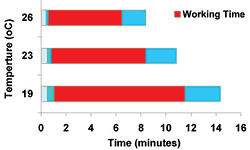
Viscosity
Viscosity is a measure of the resistance of a fluid to deformation under shear forces and is commonly described as “thickness” of a fluid. Viscosity also represents the resistance to flow and is thought to be a measure of fluid friction.
Water apparently is “thin” and has a low viscosity, whereas pitch is “thick” having a high viscosity. Solids may have viscous properties to some extent. It has been said that “a solid can be regarded to be a fluid of extremely high viscosity.” Solid bodies, such as a cement mantle, which have elasticity and viscosity in a particular range of deformation and rate of deformation, are called viscoelastic.
During the working phase, there are two requirements for bone cement viscosity — it must be sufficiently low to facilitate the delivery of the cement dough from the syringe to the bone site, and it must penetrate into the interstices of the trabecular bone.
On the other hand, the viscosity of the bone cement should be sufficiently high to withstand the back bleeding pressure, thus avoiding the risk of inclusion of blood into the cement, because this could significantly reduce the stability of the bone cement. It is important that the cement retains an optimized viscosity for an adequate duration to allow a “comfortable” working time. Pulsatile lavage is mandatory to wash the bone marrow and blood out of the intertrabecular spaces and to reduce the incidence of pulmonary embolism.9
The most important function of bone cement is to evenly transfer load from the implant to bone. Cement also acts as a shock absorber. These favorable characteristics of cement are enhanced by optimizing prosthesis geometry. Different parameters are used for testing the mechanical properties of bone cement.
Methods for testing are defined by international regulations (ISO 5833) or national standards. Tests may be static or dynamic. Cyclic loads assess fatigue strength and some failure mechanisms such as crack propagation. Usually the following static strength properties are investigated: bending strength, compressive strength, and tensile strength. Bone cements with compressive strengths of >70 MPa (1 Pa=0.00001 bar= 1 N/m2) are suitable for implant fixation.
Creep
Creep is a time-dependent, permanent deformation of a material under load. Creep is most prevalent under high stresses (and temperatures) and is not necessarily a failure mode. The creep resistance of bone cement results in reduction of the prosthesis subsidence in the cement mantle. Polished tapered stems are intended to engage with the cement as they subside under load.
In vitro experiments performed by Norman10 clearly demonstrated the relocking of tapered polished stems that occurs with loading. Reinsertion after pull out and weight bearing such as single-legged stance (SLS), stair climbing, or cyclic loading resulted in a bond strength almost equal to the initial pull-out loads.11
These results emphasized the benefit of the so-called taper lock of polished stems. A subsidence of >5 mm is thought to compromise long-term stability. Radiostereometric analyses by Kärrholm et al12 demonstrated the excellent long-term results of tapered polished stems, even in the presence of subsidence. The design and surface finish of cemented stems must be adapted to the fixation philosophy.13
The clinical reports of Sochart et al14demonstrated the outstanding long-term results that can be achieved with polished cemented stems in young patients.
Porosity
The mechanical properties and long-term stability of bone cements are affected by incorporation of air into the cement mantle, which may occur during mixing when air between the cement powder beads is enclosed into the dough. Voids may also be formed as a result of evaporation of the monomer under high temperature during polymerization. Additionally, air can be locked into the cement during its application into the bone bed. Porosity describes the ratio of non-solid volume (Vp) to the total volume (Vm) of any material.
To achieve solid fixation, all measures should be taken to minimize both the size and number of pores, as they may act as voids and compromise the stability of the cement mantle by crack initiation and crack propagation. Vacuum mixing is one of the most important methods of preventing pore formation.
Micromorphology
Histological examination of cemented implants is time consuming and difficult because embedding the specimen and cutting and (surface-) staining require a particular experience and expertise, involving techniques that are not routinely available in the laboratory.
Cutting off probes and grinding off slices need special apparatus. The so-called contact point technology for cutting the bones with cement and implant in place reduces material consumption and saves time. Contact point cutting technology aims at having a small area of contact between the probe and the saw. This results in less deformation of the probe, a high quality of the surfaces, a shorter cutting time, a better chip removal, and easier cooling conditions. Charnley3,15 was one of the first to describe the tissue reactions of the surrounding bone induced by PMMA.
Charnley emphasized the presence of load bearing, intimate bone contact with the cement surface, connective tissue, and some sporadic foreign body giant cells. Willert et al16,17 proposed a time-dependent grading of the tissue reaction and identified three phases: initial phase, reparation phase, and stabilization phase.
The initial phase is a reaction to the surgical trauma due to sawing, rasping, broaching, and the contact of the cement. It is characterized by necrosis in the bone and the marrow. After the removal of this debris, the reparation phase starts with a synthesis of collagen yielding a thin membrane consisting of connective tissue.
At the base of this membrane, new bone formation can be observed. During the phase of stabilization, more bone is produced, mainly in the part of the membrane opposite the cement. In some regions, osteoid and non-calcifying bone can be found. Synovial cell-like membranes sometimes occur, and these cubic cells are assumed to become macrophages.
Even after comparatively long periods of implantation, there is a permanent and close contact of bone to the cement. Small trabeculae can survive embedded into a huge mass of PMMA. Mechanically, the spongy bone is reinforced by the acrylic resin. Osteoblasts, or lining cells, are only rarely observed on the surface of bone.
However, even following long periods of implantation, intimate contact is observed and the bone is viable. Some zones of non-calcified bone can be found. These areas are regarded as consisting of osteoid, but osteoblasts can be difficult to find. Some authors have described these sites as lines of disturbed mineralization due to bleeding of the toxic DMPT out of the cement.
The author believes that these sections of the bone are mostly connective tissue that is part of an existing interface. In retrievals with unimpaired function and stability of the implant, intimate bone/cement fixation alternates with areas of connective tissue. Debris particles are either encapsulated by membranes and foreign body giant cells or incorporated by macrophages.
Antibiotics
In the early 1970s, there was an infection rate of >7%, which led Buchholz et al18 to add antibiotics to the bone cement. The aminoglycosides proved to be the best and most practical antibiotics, not only in view of their antibiotic spectrum but also due to their chemical properties.
Gentamicin (Figure 19) is a molecule that exhibits extreme stability during sterilization procedures, manufacturing processes, and its shelf life. It is resistant to the temperatures that occur during the polymerization process, and it is readily delivered from the polymers and acts locally in high concentrations without any systemic adverse effects. Buchholz18 reduced the infection rate by >6%.
A tendency exists, especially in Europe, to use antibiotic-loaded cement exclusively in both primary and revision hip arthroplasty. Certain bacteria adhere to the surface of synthetic and metallic materials and protect themselves by the production of a slime.
In this so-called biofilm, which is a glycocalyx consisting of polysaccharides, bacteria are shielded from both the hosts’ immunoreaction and attack by antibiotics. Biofilms are a special kind of hydrogels and are characterized by small canaliculi that serve as nutrition canals and channels for the transfer of messages (“quorum sensing”).
Bacteria in a biofilm usually reveal a resistance against the common antibiotics. The worst bacteria are methicillin-resistant Staphylococcus aureus and methicillin-resistant Staphylococcus epidermidis.
There is considerable evidence that the probability of formation of a biofilm is reduced in the vicinity of antibiotic-loaded acrylic cement. In the early postoperative course (10 hrs), there is a high elution rate with >30% of the ultimate dose eluted. During the next 2 weeks, in a second and slower elution phase, 60% is released, and only 10% is washed out during a third phase until 10 weeks postoperatively. The release of antibiotics has little influence on the mechanical properties of cement, especially the fatigue strength.
Care must especially be taken when other antibiotics are added to bone cement because physico-chemical and mechanical properties may be altered and may induce detrimental effects in long-term stability, especially when aqueous solutions are applied. Some antibiotics even influence the setting time.
Future
Alternative antibiotic and antiseptic agents will be provided by manufacturers in the near future. There will also be more sophisticated technical assistance to guide the timing of insertion of the cement, but no technology will replace knowledge and experience. Education and training will remain the cornerstone for optimized cement handling and application. Bone cement is the only implant that is manufactured in the operating room and must meet the high standards demanded of any other implant supplied by the industry.
References
- Gluck T. Referat über die durch das moderne chirurgische Experiment gewonnenen positiven Resultate, betreffend die Naht und den Ersatz von Defecten höherer Gewebe, sowie über die Verwerthung resorbirbarer und lebendiger Tampons in der Chirurgie. Archiv Klin Chir. 1891; 41:187-239.
- Charnley J. Anchorage of the femoral head prostheses of the shaft of the femur. J Bone Joint Surg Br. 1960; 42:28-30.
- Charnley J. Acrylic Cement in Orthopaedic Surgery. Baltimore, Md: Williams and Wilkins; 1970.
- Biehl G, Harms J, Hanser U. Experimentelle Untersuchungen über die Wärmeentwicklung im Knochen bei der Polymerisation von Knochenzement. Unfall Chir. 1974; 78:62-69.
- Tosvig-Larsen S, Franzen H, Ryd L. Cement interface temperature in hip arthroplasty. Acta Orthop Scand. 1991; 62:102-105.
- Kirschner P. Experimentelle Untersuchungen mechanischer und chemischer Eigenschaften von Knochenzementen nach Langzeitimplantation im menschlichen Körper. Habilitationsschrift, Mainz; 1978.
- Schlag G. Experimentelle und klinische Unetrsuchungen mit Knochenzementen. 1974; Hollinek, Wien.
- Breusch SJ, Kühn KD. Knochenzemente auf der Basis von Polymethylmethacrylat. Orthopäde. 2003; 32:41-50.
- Pitto RP, Koessler M, Kuehle JW. Comparison of fixation of the femoral component without cement and fixation with the use of a bone-vacuum cementing technique for the prevention of fat embolism during total hip arthroplasty. J Bone Joint Surg Am. 1999; 81:831-843.
- Norman TL. Effect of PMMA creep and prosthesis surface finish on the behaviour of a tapered cemented total hip stem. In: Walenkamp G, Murray DW (eds). Bone Cements and Cementing Technique. Springer, Berlin; 2001; 183-189.
- Hustosky KT, Norman TL, Kish VL, Gruen TA, Blaha JD. The effect of surface finish on the behavior of tapered cementetd total hip stems. American Society of Biomechanics 23rd Annual Meeting; 1999.
- Kärrholm J, Nivbrant B, Thanner J, et al. Radiostereometric evaluation of hip implant design and surface finish. Scientific Exhibition. 67th Annual Meeting of the American Academy of Orthopaedic Surgeons; 2000.
- Dall DM, Learmonth ID, Solomon MI, Miles AW, Davenport JM. Fracture and loosening of Charnley femoral stems. Comprison between first-generation and subsequent designs. J Bone Joint Surg Br. 1993; 75:259-265.
- Sochart DH, Porter ML. Long-term results of cemented Charnley low-friction arthroplasty in patients aged less than 30 years. J Arthroplasty. 1998; 13:123-131.Charnley J. The reaction of bone to self-curing acrylic cement. A long-term histological study in man. J Bone Joint Surg Br. 1970; 52: 340-353.
- Willert HG, Puls P. Die Reaktion des Knochens auf Knochenzement bei der Allo-Arthroplastik der Hüfte. Arch Orthop Unfallchir. 1972; 72:33-71.
- Willert HG, Ludwig J, Semlitsch M. Reaction of bone to methacrylate after hip arthroplasty: a long-term gross, light microscopic, and scanning electron microscopic study. J Bone Joint Surg Am. 1974; 56:1368-1382.
- Buchholz HW, Elson RA, Engelbrecht E, Lodenkämper H, Röttger J, Siegel A. Management of deep infection of total hip replacement. J Bone Joint Surg Br. 1981; 63: 342-353